What do soil surfaces look like to absorb 200mm rainfall per hour? This question is not asked in New Zealand agriculture and is overlooked because no text book illustrates this essential principle. The photo shows what soil surfaces look like to maximise rainfall absorption – cottage cheese, not cheddar. Note the soil colour is black, not grey as many NZ farming soils are described. This colour, and it’s associated absorption properties, are due to organic matter and the amount of one element: carbon.
Independent international researchers like Australian Dr Christine Jones and American Dr Elaine Ingham have been spearheading interest in soil carbon. They have inspired farmers to challenge practices and try new production methods resulting in reduced inputs and lower production costs. Likewise, North American Gabe Brown and Australian Col Sies are proving changes in farming practices are delivering a range of production, environmental and financial benefits but scientists debate its accuracy and relevance. Despite controversy, more farmers willing try these ideas and remark on benefits they observe. Even UK dairy farmers are challenging high inputs systems to grow soil carbon.
Soil carbon is precious for farmers. It determines how much water soil can hold, the fertility and biodiversity present in the soil, and therefore the length of growing seasons. Every kg of carbon holds at least 4kg of water and a lift of 1% soil carbon in top 10cm is equivalent of Aus$1,500 – Aus$2,000 with current fertiliser prices. (The bank we draw nutrients from) High carbon, biologically active soils are often two degrees warmer than those which aren’t. It’s said this is the equivalent of dropping over 300 metres in altitude.
So what is the potential of soil carbon? At the Paris summit, New Zealand signed up to the French government initiative to sequester 4% of carbon in soil to improve soil organic matter content globally. Why do that? Because soil holds more carbon than living plants and atmosphere combined. The problem is the ever changing amounts present in soils, is primarily due to what is happening on soil surfaces and above.
Photosynthesis drives soil carbon with 10-40% of photosynthesised carbon passing through plant roots within an hour (Kumar et al, 2006). These exudates comprise of more than 150 compounds which feed soil organisms (Narasimhan et al, 2003). It is activities of bacteria, fungi and other soil life which stick together soil particles into aggregates that create soil structure. The nature of carbon compounds determines whether it’s labile (and quickly decomposed) or recalcitrant, the ideal forms for sequestration.
Farmers and agricultural professionals are excited about possibilities here, so why isn’t more said? Clearly passionate about soil carbon, Louis Schipper, a professor at the University of Waikato’s Earth and Ocean Science department, observes that there is a lot of noise but not hard data on what pastoral practices sequester and lose carbon in New Zealand and elsewhere (Schipper et al, 2017). Schipper suggests that surprisingly little is known because nobody thought of looking at soil carbon. Instead most soil analysis focused on minerals for fertiliser companies.
Establishing trends around soil data is compromised by time periods between sampling (often inconsistent timing) and sample depth (many samples are only taken to a depth of 10cm when deeper layers offer more carbon storage potential). Furthermore, different soil types which have differing structural capacities to store carbon and soil bulk density (amount of air in soil) which lowers carbon storage as soils become more dense (less air).
The Schipper review makes clear phosphate fertiliser doesn’t make any difference to carbon stocks. Irrigation while increasing production reduces carbon levels most likely due to higher soil microbial activity. There is no definitive data yet on the effects of nitrogen, or the impact of earthworms. We know pasture renewal loses carbon, up to 1-2 tonne depending on whether or not cultivating or direct drilling is used (due to decomposing roots and soil biology). Bare soil surfaces exacerbate the amount lost, therefore direct drilling reduces the amount lost compared to cultivation. Technologies like bio-char and carbon based soil conditioners affect soil carbon but they are merely surface amendments.
Forest soils have less carbon than pastures meaning when pastures replace forests soil carbon levels rise. However, Schipper believes it’s unlikely the total carbon per hectare increases when trees are replaced by pasture.
Surprisingly, what isn’t known is how grazing regimes affect soil carbon levels in New Zealand. Instead, Schipper focuses on farming systems (dairy, dryland, irrigated, hill country) and ignores grazing regimes. Grazing has greatest influence on sward composition, amount of litter on soil surfaces, size of photosynthetic leaf area, and therefore extent of exudates pumped into the soil. No effort has been made to look at the timing of grazing, grazing frequency and pasture recovery times, nor stock density (as compared to carrying capacity). Instead most scientific interventions focus on expensive technologies such as irrigation, synthetic fertilisers, pharmaceuticals, and biochar and when are their carbon footprints ever evaluated?
Grasslands and pastures tend to have a higher proportion of root mass in the top 60cm of soil than trees (Schrumpf et al, 2013) and grazing regimes have a greater influence on root biomass and therefore rhizosphere volume and depth than abandoned pastures (Hafner et al, 2011). Root growth increases root exudates (Badri & Vivanco, 2009, Schrumpf et al, 2013; Nguyen, 2003) meaning as plants recover from grazing, and increase leaf surface area, they pump more exudates into soil.
Exudates volume declines with root age meaning secretions are not homogeneous along the root length with more activity at the root tip (Walker et al, 2003). Growing roots pump carbon compounds into the soil allowing easier passage. Pulse grazing techniques encourage this phenomenon.
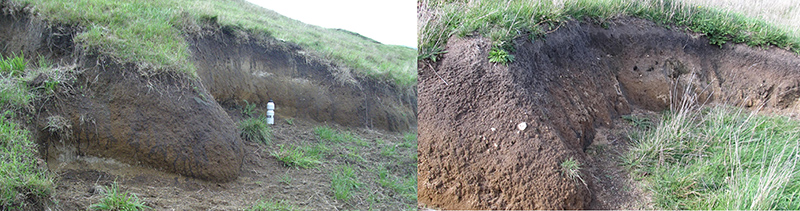
(Soil change over four years) The same bank four years apart showing how plant roots help develop soil. Black streaks follow roots down into pumice soil in 2011 (left photo) extending the A horizon. By 2016 the white pumice had gone replaced by a deeper A horizon.
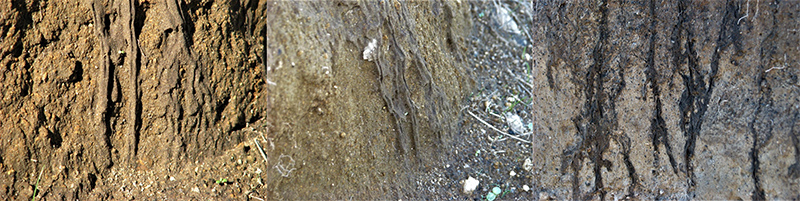
(Roots and soil carbon) Various photos demonstrating how roots create soil in rhizosphere (root zone) compared to soil surrounding it by pumping exudates and decomposing dead roots.
While much research draws from grasses and pasture plants growing through life stages under scientific conditions, how this differs from pulsing mature plants under grazing conditions as found on farms, is where there is more work to do. Could observations of why diverse pastures hold carbon levels steady, whereas ryegrass monocultures lose carbon, be due to unrealistic productivity claims about monocultures? And does this then drive overgrazing and stripping of ryegrass plant abilities to regenerate themselves and feed their soil communities?
Where are New Zealand scientists targeting their focus? There are three areas; hill country, tussock grasslands, and peat soils. Hill country data is showing increases in soil carbon and scientists aren’t sure why. Tussock grasslands which suffer from overgrazing, burning, and pests can lose carbon but proper grazing including occasional fallow from grazing is good for soil carbon. Both these situations could reflect how grazing practices influence soil carbon levels.
Peat soils notoriously lose carbon. Found in Northland, Waikato and Southland, they can be 9-10 metres deep. Once drained they can lose 3-4 tonne per year and while it might take 100 years or longer for those soils to fade away, all that carbon is going into the atmosphere. The question to be asked here is where drainage is required and whether rates of decomposition can be slowed as higher water tables prevent decomposition.
However, long time soil carbon advocate, and ex-Agmardt board member, Waikato farmer Max Purnell contests many issues around current New Zealand soil carbon research. He argues scientists do not look deep enough, at least a metre deep, to see where much higher rates of carbon accumulation occur. He cites private research showing highest rates of carbon accumulation happen anywhere between 1 to 6 metres. This conflicts with the review on carbon sequestration rates and potential soil volumes because few New Zealand academics have looked this deep.
Nowhere in the Schipper review is the nature of carbon measured defined in New Zealand research. Are they short or long chain carbon compounds? There is recognition that shorter chain molecules are more labile and predisposed to decomposition. Longer chain carbon molecules are more stable than shorter and it’s claimed these are deposited in greater qualities in deeper soil profiles. Schipper doubts this. Soil carbon is considered more stable at depth due to increased residence time and declining mineralisation rates meaning carbon is less labile and more recalcitrant (Schrumpf et al, 2013). However, some researchers assert fresh carbon entering deeper soil layers decomposes recalcitrant soil carbon by stimulating dormant microbes but have yet to prove this theory at depth in soil (Fontaine et al, 2007).
Getting real data from deep soil profiles is difficult and expensive. There is recent work being demonstrated in Canada where soil cores to a metre deep are being examined and similar work is happening in New Zealand. Dr. Christine Jones’s private research highlights greater change in carbon levels deeper down the soil profile on an Australian farm but New Zealand science has yet to document this.
Furthermore, there are few farmers in New Zealand who graze to increase soil carbon as their grazing focus is livestock performance not ecosystem enhancement.
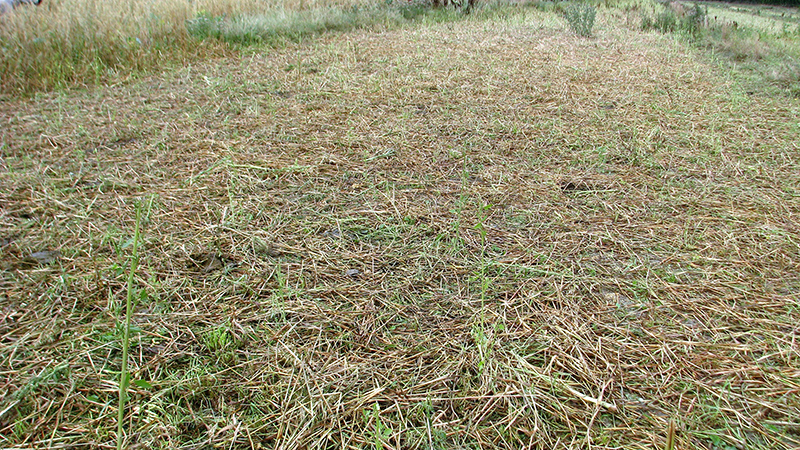
(Cover crop grazing) This amount of litter is perceived as wastage forage in modern farming. Few farmers plan grazing to build soil structure or enhance soil function. Grazing to build soil function is overlooked or ignored, especially if farmers are financially strained.
One successful technique, sabbatical fallow, is explored by Mackay et al (1991) whereas Golding et al (2011) show deeper rooting plants promote higher growth rates in livestock. Both have potential to lift carbon levels. Teague et al (2016, 2013) explore grazing and agroecology with great clarity but it’s unclear whether New Zealand researchers are truly motivated given the general encouragement to explore technology based solutions and modelling programmes.
It’s this lack of real soil carbon data at depth that adds to why Purnell believes steady state modelling founding soil carbon predictions are flawed. He argues these models are conceptually based rather than relying on actual measurements, particularly below 30cms. Therefore, establishing relationships on modelled data to predict soil carbon levels further down soil profiles is speculative, something both Goh (2004) and Schipper et al, (2017) acknowledge. It highlights frustration between soil carbon promoters and a scientific community who understand physical and chemical mechanisms of soil carbon stabilisation and acknowledge their limitations regarding biological mechanisms.
Purnell is not alone in his criticism of modelling programmes guiding environmental advice and relationships to soil carbon. As independent soil scientist Samuel Dennis points out, a divisive issue for farmers is the relationship between Overseer, the nutrient budgeting programme driving the basis of productivity limits for many regional councils, and soil organic carbon and nitrogen. Overseer predicts nitrogen losses from farming systems, but carbon and nitrogen are intricately linked, so whenever carbon is accumulating in soils nitrogen is accumulating also, reducing nitrogen losses.
However, nitrogen accumulation rates can only be adjusted crudely in Overseer, and users are advised not to adjust them at all as the model is not calibrated for such changes. Overseer doesn’t recognise factors contributing to C and N accumulation such as surface pasture litter, volumes of exudates leaving plant roots, or root depth.
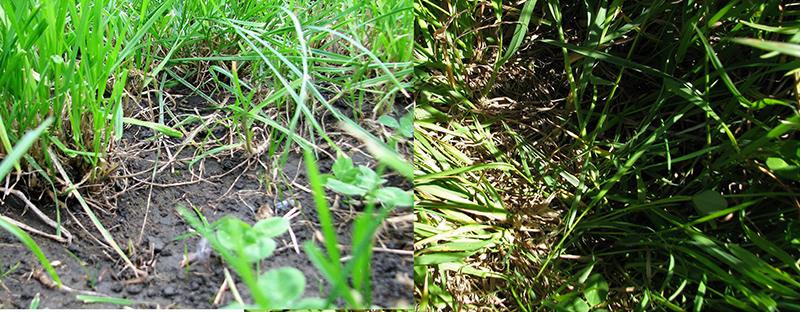
(Litter verses Bare Soil) Spot the difference? Overseer can’t. Good soil building practices employed by farmers are not recognised by Overseer or its operators. Overseer predicts nitrogen leaching but ignores practices which build soil capacity to hold and prevent nitrogen leaching.
These attributes have a strong bearing on soil abilities to deal with nitrogen leaching. So it doesn’t reward farmers who are building capacity to hold nitrogen.
So where does this leave the soil carbon debate? Schipper’s view is clear; that carbon credits are unlikely incentives. He explains if a 100ha property sequestering 1 tonne C/ha/yr; that is 100 tonne per year for the property. If price per tonne of carbon was $NZ25/tonne (currently $17) the whole farm would raise only $2,500. Add on costs of measuring soil carbon or a lower price if carbon is lost throughout the year and net income will be less. While sequestration rates might be high initially they cannot be maintained as carbon levels rise. Based on this thinking there is no carrot here.
Therefore, current science belief is while soil carbon is good for farming, farmers will make more money doing other things than sequestering carbon because market price and environmental processes restrict potential to create reasonable income with carbon credits. Compounding this situation is domineering technology like Overseer which doesn’t encourage farmers to build soil health, just manipulate their inputs. This reflects current scientific endeavours focusing on production based intellectual property; agri-chemicals, equipment, and technical processes and pathways, rather than applying cheaper and more sustainable agroecology principles to address climate change, environmental health, and farm profitability.
Yet despite conflicting views, both sides believe the greatest advantages for farmers increasing soil carbon, come from improved soil health; the emerging term which emphasises greater water absorption and retention and higher fertility. Amounts, rates, and nature of these benefits will be the focus of further debate. What is not being denied is that soil carbon can reshape the nature of agricultural production through enhancing landscape function and community resilience.
References
Badri, D. V. and Vivanco J. M. (2009). Regulation and Function of Roots Exudates. Plant, Cell, and Environment 32, 666-681.
Fontaine, S., Barot, S., Barre´, P., Bdioui, N., Mary, B. & Rumpel, C. (2007). Stability of organic carbon in deep soil layers controlled by fresh carbon supply. Nature 450, 277-280. DOI: 10.1038/nature06275
Goh, K. M. (2004). Carbon Sequestration and Stabilisation is Soils. Implications for Soil Productivity and Climate Change. Soil Science Plant Nutrition, 50 (4), 467-476
Golding, K.P., Wilson, E.D., Kemp, P.D., Pain, S.J., Kenyon, P.R., Morris, S.T., and Hutton, P.G. (2011). Mixed Herb and Legume Pastures Improves growth Rates of Lambs Post Weaning. Animal Production Science 51 717-723
Hafner, S., Unteregelsbacher, S., Seeber, E., Lena, B., Xu, X., Li, X., Guggenberger, G., Miehe, G., and Kuzyakov, Y. (2011). Effect of grazing on carbon stocks and assimilate
partitioning in a Tibetan montane pasture revealed by 13CO2 pulse labelling. Global Change Biology, doi: 10.1111/j.1365-2486.2011.02557.x
Kumar, R. Pandey, S. and Pandey, A. (2006). Plant Roots and Carbon Sequestration. Current Science 91 (7) 885-890.
Mackay, A. D. Budding, P.J. Ross, D.J. Tate, K.R. Orchard, V.A. Hart, P.B.S Kettles, H. A. (1991). Pastoral Fallow for Improving Low Fertility Hill Country Pastures. Proc NZ Grassland Assoc 53 209-213
Narasimhan, K. Basheer, C. Bajic, V. B. and Swarup, S. (2003). Enhancement of Plant-Microbe Interactions Using a Rhizosphere Metabolomics-Driven Approach and Its Application in the Removal of Polychlorinated Biphenyls. Plant Physiology 132, 146-153.
Nguyen, C. (2003). Rhizodeposition of organic C by plants: mechanisms and controls. Agronomie 23 375–396.
Schipper, L A, Mudge, P, L., Kirschbaum, M. U. F, Hedley, C. B., Golubiewski, N. E., Smaill S. J., and Kelliher, F. M (2017): A Review of Soil Carbon change in New Zealand’s Grazed Grasslands. New Zealand Journal of Agricultural Research, DOI: 10.1080/00288233.2017.1284134
Schrumpf, M., Kaiser, K., Guggenberger, G., Persson, T., Kogel-Knabner I., and Schulze E.-D. (2013). Storage and stability of organic carbon in soils as related to depth, occlusion within aggregates, and attachment to minerals. Biogeosciences, 10, 1675-1691
Teague, W.R., Apfelbaum, S., Lal, R., Kreuter, U.P., Rowntree, J Davies, C.A., Conser, R., Rasmussen, M., Hatfild, J., Wang, T., Wang, F., and Byck, P. (2016) The role of ruminants in reducing agriculture’s carbon footprint in North America. Journal of Soil and Water Conservation, 71 (2) 156-164
Teague, R., Provenza, F., Kreuter, U., Steffens, T., Barnes, M. (2013). Multi-paddock grazing on rangelands: Why the perceptual dichotomy between research results and rancher experience? Journal of Environmental Management 128, 699-717
Walker, T. S., Pal Bais, H., Grotewold, E., and Vivanco, J. M. (2003). Root Exudation and Rhizosphere Biology. Plant Physiology 132, 44-51
Leave a comment